Get daily insight, inspiration and deals in your inbox
Get the hottest deals available in your inbox plus news, reviews, opinion, analysis and more from the TechRadar team.
Explore TechRadar
Reviews
All reviews-
Aura Carver Mat review: a gorgeous but flawed digital photo frame
This 10-inch digital photo frame by Aura looks the part and is super easy to use – but it isn't perfect
-
-
Ricoh GR IIIx review
The Ricoh GR IIIx offers a new twist on the GR III, thanks to its 40mm f/2.8 lens. Does this make it the ultimate street shooter?
-
Sony Cyber-shot RX100 VII review
The RX100 VII delivers great images, crisp videos and stellar AF performance, but it's hard to get past the price tag.
-
Ninja Double Stack air fryer review: half the footprint, double the fun
Ninja's innovative Double Stack air fryer is designed for those with small kitchens but big appetites, and it really delivers.
-
reMarkable 2 review: still the best e-paper note-taking device money can buy
If you love to sketch and jot down notes, there’s no better e-paper tablet than the reMarkable 2, but that’s all it’s good for.
-
iPad Air 13-inch (2024): You no longer need to go Pro
With the iPad Air (2024), Apple adds all new size. Here's our first impressions of the 11-inch and 13-inch iPad Air with M2.
-
Fujifilm Instax mini 99 review: Instant fun!
The Instax mini 99 is an exciting new option for fans of film photography who want to get creative.
-
How TechRadar tests
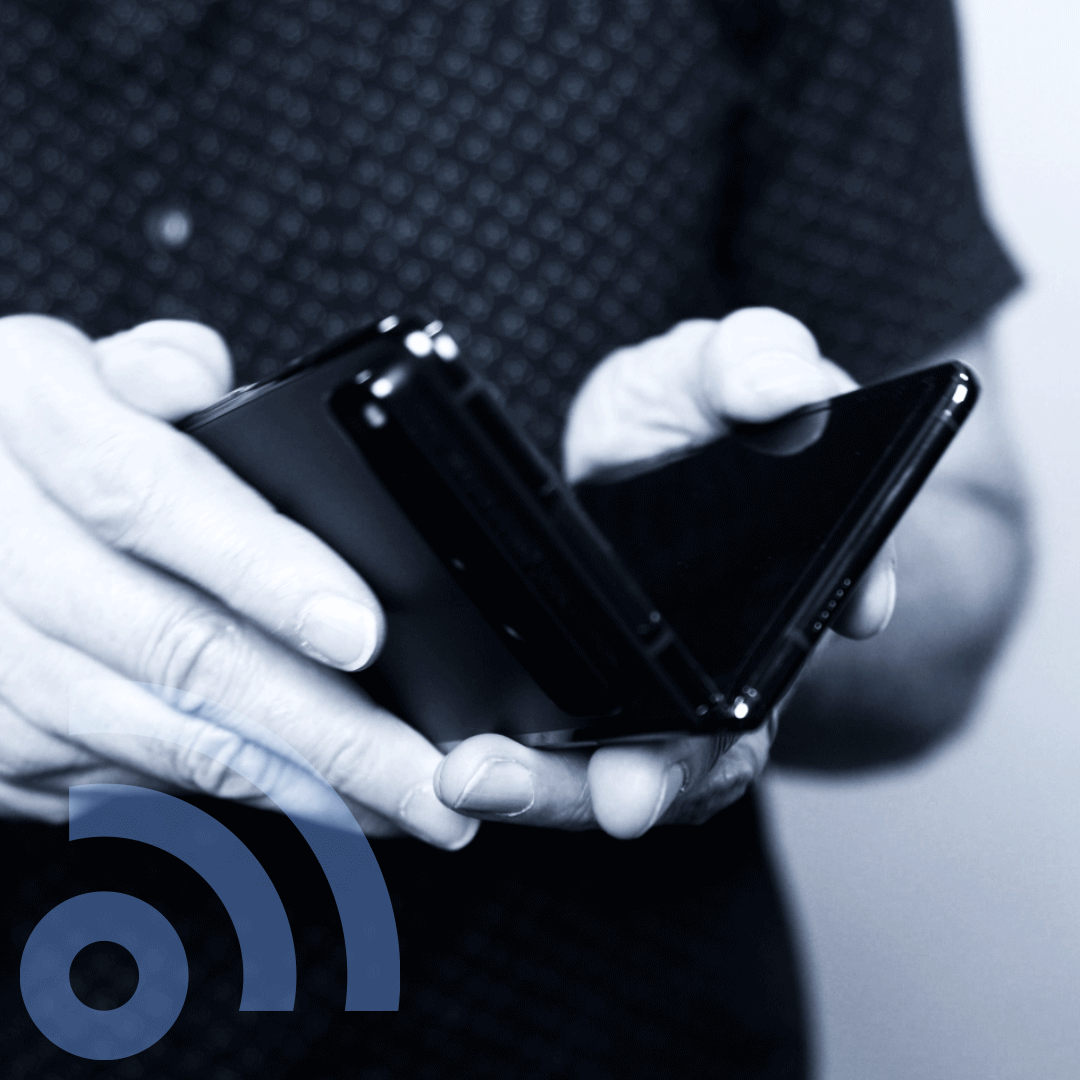
Product testing for the real world
You need to know that the device or service you’re about to spend money on works as advertised - and that it works in the real world.
- We test properly: objective and subjective testing
- We use experienced experts for our reviews
- We always offer 100 per cent unbiased, independent opinions
reviews
hours' testing
buying guides
Phones
All Phones-
Select iPhones can imitate Google's handy Circle to Search tool with new shortcut
Google developers have launched Screen Your Screenshot for the iPhone 15 Pro, but it may actually work on older models.
-
-
Updated
The best phone 2024
We've tested the best phones around, in every price range, to bring you this definitive ranking of the best smartphone to buy.
-
Updated for 2024
The best prepaid phone plans for May 2024
Looking to save some cash on your cell phone bill? See our list of this month's best prepaid phone plans to get some top recommendations.
-
Get free maps, emails, and rideshare nationwide with TextNow
TextNow has extended its exceptional free service to include essential apps like maps, emails, and Uber.
-
The Google Pixel 8a is here - and yes, you can already get it for free with this deal
The latest Google Pixel 8a offers a compelling array of specs for a wallet-friendly mid-range price.
-
Quick! The Google Pixel 8 Pro is $440 off at Mint Mobile right now - the lowest price yet
This Pixel 8 Pro deal at Mint Mobile won't last - it's the cheapest upfront price yet without the need for a pesky trade-in.
-
The iPhone 17 line could include huge upgrades and a new Slim model
A smaller Dynamic Island, a more complex design, and loads of RAM could all be planned for the iPhone 17 series.
-
Laptops & Computing
All Laptops & Computing-
Do nearly all Indian men wear turbans? Generative AIs seem to think so, and it’s only the tip of the AI bias iceberg
Giving almost all Indian men turbans and other racist prompt results — can AI get past its bias stumbling block?
-
-
Logic Pro 2 is a reminder that Apple's AI ambitions aren't just about chatbots
The next-release of Logic Pro for iPad shows off Apple's AI chops with three new features.
-
The new Magic Keyboard and Apple Pencil Pro look good, but Apple urgently needs to revisit its single worst accessory
Forget the new Magic Keyboard and Apple Pencil Pro - there’s only one Apple accessory in dire need of a redesign.
-
Tired of ads in Windows 11? This free, straightforward third-party app might be just what you need to cut down the number of ads on your PC
Fed up with Windows 11 ads? OFGB could be your solution! It's a free app for disabling various ads across the operating system, providing a cleaner, hassle-free experience.
-
OpenAI is working on a new tool to help you spot AI-generated images and protect you from deep fakes
An AI image-spotting tool is in the works from OpenAI, and it's impressive in some respects, with a notable catch.
-
The best laptop deals in May 2024
We've gathered up all of the best laptop deals available now featuring a range of devices to suit multiple needs and budgets.
-
Intel gives consumers advice on high-end Core i9 CPUs that are crashing – and it’s about time
If you’re puzzled after reading Intel’s advice, don’t worry – we’re here to cut through the confusion.
-
Apple
All Apple-
Updated
The best phone 2024
We've tested the best phones around, in every price range, to bring you this definitive ranking of the best smartphone to buy.
-
-
Logic Pro 2 is a reminder that Apple's AI ambitions aren't just about chatbots
The next-release of Logic Pro for iPad shows off Apple's AI chops with three new features.
-
The new Magic Keyboard and Apple Pencil Pro look good, but Apple urgently needs to revisit its single worst accessory
Forget the new Magic Keyboard and Apple Pencil Pro - there’s only one Apple accessory in dire need of a redesign.
-
The Apple M4 chip’s AI performance just leaked – and it might not be what you expected
A set of leaked Geekbench results appear to show the Apple M4 chip in action. How well does it perform?
-
The Apple AirPods are down to their super-cheap Black Friday price at Best Buy
The Apple AirPods 2 aren't the newest earbuds but they're still great for Apple owners – and they're now on sale at a great price.
-
Streaming
All Streaming-
Kingdom of the Planet of the Apes proves there’s still life in this decades-old franchise
With its downright jaw-dropping visual effects and surprisingly compelling story, Kingdom of the Planet of the Apes is a worthy entry in this long-running franchise.
-
-
Updated
The Boys season 4: Prime Video release date, trailers, cast, plot synopsis, and more
Here's what we know about The Boys season 4 ahead of its mid-June debut on Prime Video.
-
Updated
Disney Plus price: subscriptions available in 2024 worldwide
A dash of fairy dust ought to get the right Disney Plus price for you. Here's your complete guide to all the latest subscription rates.
-
Prime Video movie of the day: Top Gun Maverick is an infinitely rewatchable thrill ride
The sequel to the iconic Air Force movie is as much fun as the original, and the flight scenes are spectacular.
-
Techradar deals
Hulu with Live TV explained: price, plans, and channels
Stream more than 95 channels live around the States on top of its on-demand library with the Hulu with Live TV package. Here's everything you need to know.
-
TVs
All TVs-
-
Hot deals
The cheapest OLED TV deals and sales for May 2024
Your guide to the best OLED TV deals with incredible sales from brands like Sony, LG, and more.
-
Updated
The best 65-inch TV 2024
We pick out the best 65-inch 4K TVs to complete your home theater setup, no matter how much you want to spend.
-
The best Mother's Day gift ever: Samsung's The Frame TV drops to a record-low price
Samsung's gorgeous The Frame TV might just be the best Mother's Day gift ever and it's on sale for a new record-low price.
-
The best 4K Blu-ray players 2024
Streaming services are popular, but there's no better way to watch movies than with the best 4K Blu-ray players.
-
Updated
The best 75-inch TVs 2024
The best 75-inch TVs offer a true big-screen experience, with a size befitting the greatest films and the most nail-biting sports matches.
-
Updated
The best soundbars for 2024
From budget-friendly bars to Dolby Atmos wonders, the best soundbars make it easy to get a serious TV audio upgrade.
-
Samsung's new, cheaper OLED TVs are now available to buy
Samsung's entry-level S85D series has arrived, providing a less expensive OLED TV option that still offers premium features.
-
Audio
All Audio-
-
Best Apple Memorial Day sales 2024: today's best early deals
This is your 2024 Memorial Day Apple sales guide, with everything you need to know and today's best Apple deals.
-
The leaked Sonos Roam 2 speaker design looks awfully familiar
The upcoming Sonos Roam 2 looks very similar to its predecessor, bar one useful upgrade.
-
Updated
The best Bluetooth speakers 2024
Looking for the best Bluetooth speaker money can buy? Here are the top portable speakers on the market right now.
-
The slicker new Sonos app is available as a free upgrade now
Download and open it before you need it, because it'll need to check your system first.
-
The Apple AirPods are down to their super-cheap Black Friday price at Best Buy
The Apple AirPods 2 aren't the newest earbuds but they're still great for Apple owners – and they're now on sale at a great price.
-
Final's new planar magnetic headphones are dark, moody, wired… and oh-so expensive
The elite new D7000 headphones use 'Air Film Damping' to solve the issue of bass registers in planar magnetic cans.
-
Hot deals
The best Sony WH-1000XM5 deals, prices and sales for May 2024
We're rounding up all the best Sony WH-1000XM5 deals with all the best prices around the web for Sony's noise canceling headphones.
-
Health & Fitness
All Health & Fitness-
-
This new temperature-regulating, snore-detecting mattress cover definitely wasn’t on my 2024 bingo card
Two years after launching the Pod 3 smart mattress cover, Eight Sleep are back with new Pod 4 and Pod 4 Ultra models
-
What is Hyrox? The trending fitness race explained, plus all the gear you’ll need
What a Hyrox race is, why you should do one and the gear you’ll need to succeed.
-
Samsung Galaxy Watches (and the Galaxy Ring) could get an AI-powered heart health upgrade
Samsung's latest patent could be big news for anyone wanting to take a more comprehensive picture of their heart health.
-
Cameras
All Cameras-
-
Our favorite Sony camera has a massive $400 off in this cashback deal
Sony rebates have knocked $400 off the Sony A7R V, $300 off the Sony ZV-E1 and $200 off the Sony A7 IV at leading retailers.
-
Ricoh GR IIIx review
The Ricoh GR IIIx offers a new twist on the GR III, thanks to its 40mm f/2.8 lens. Does this make it the ultimate street shooter?
-
Sony Cyber-shot RX100 VII review
The RX100 VII delivers great images, crisp videos and stellar AF performance, but it's hard to get past the price tag.
-
Updated
Best full-frame camera 2024: the top big sensor DSLRs and mirrorless cameras
Our best full-frame camera guide will help you find the perfect DSLR or mirrorless camera for you, whatever your budget.
-
Home
All Home-
-
Aura Carver Mat review: a gorgeous but flawed digital photo frame
This 10-inch digital photo frame by Aura looks the part and is super easy to use – but it isn't perfect
-
Updated
The best robot vacuum 2024
Your guide to the top robot vacuums (and mops) for hassle-free cleaning, as recommended by experienced reviewers.
-
Ninja Double Stack air fryer review: half the footprint, double the fun
Ninja's innovative Double Stack air fryer is designed for those with small kitchens but big appetites, and it really delivers.
-
TechRadar Deals
The best Keurig coffee maker sales and deals for May 2024
Save money with our guide to the best Keurig coffee maker sales and deals that area happening online.
-
Buying guides
All Buying Guides-
Updated
The best phone 2024
We've tested the best phones around, in every price range, to bring you this definitive ranking of the best smartphone to buy.
-
-
Updated
The best Kindle 2024
The best Kindle device from Amazon will help you read ebooks and even take notes or listen to audiobooks with ease
-
Updated
The best Bluetooth speakers 2024
Looking for the best Bluetooth speaker money can buy? Here are the top portable speakers on the market right now.
-
Updated
The best robot vacuum 2024
Your guide to the top robot vacuums (and mops) for hassle-free cleaning, as recommended by experienced reviewers.
-
Updated
Best full-frame camera 2024: the top big sensor DSLRs and mirrorless cameras
Our best full-frame camera guide will help you find the perfect DSLR or mirrorless camera for you, whatever your budget.
-
Updated
The best tablet 2024
The best tablets come from Apple, Samsung, and OnePlus, and each has strengths that make it a better tablet for your needs.
-
Updated
The best 65-inch TV 2024
We pick out the best 65-inch 4K TVs to complete your home theater setup, no matter how much you want to spend.
-
Why we're experts
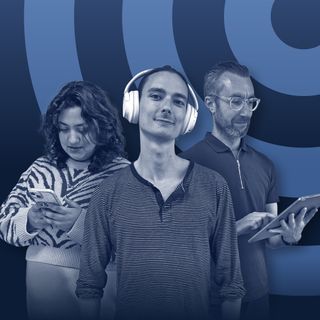
We care passionately about tech
The TechRadar team has a life-long passion for the latest innovations – over 300 years of experience between us, in fact – and we’ve made it our mission to share that combined knowledge and expertise with you.
We’re here to provide an independent voice that cuts through all the noise to inspire, inform and entertain you; ensuring you get maximum enjoyment from your tech at all times. Technology is our passion, so let us be your expert guide.
years' experience
how-tos written
Apple events covered
Deals
All Deals-
-
Best Apple Memorial Day sales 2024: today's best early deals
This is your 2024 Memorial Day Apple sales guide, with everything you need to know and today's best Apple deals.
-
Updated for 2024
The best prepaid phone plans for May 2024
Looking to save some cash on your cell phone bill? See our list of this month's best prepaid phone plans to get some top recommendations.
-
The best laptop deals in May 2024
We've gathered up all of the best laptop deals available now featuring a range of devices to suit multiple needs and budgets.
-
Our favorite Sony camera has a massive $400 off in this cashback deal
Sony rebates have knocked $400 off the Sony A7R V, $300 off the Sony ZV-E1 and $200 off the Sony A7 IV at leading retailers.
-
Updated
Disney Plus price: subscriptions available in 2024 worldwide
A dash of fairy dust ought to get the right Disney Plus price for you. Here's your complete guide to all the latest subscription rates.
-
Techradar deals
Hulu plans and prices: best deals, bundles in 2024, and how to sign up
Hop over to Hulu to find the best plans and prices for your viewing needs! Our complete guide includes bundle deals and comparisons to other streaming platforms.
-
-
-
OnePlus Coupons for May 2024
Use these OnePlus coupons to get a better price on mobiles & accessories from the leading Android smartphone retailer.
-
Google Workspace Coupon Codes for May 2024
These Google Workspace coupon codes are here so you can make bigger savings on your plan.
-
Casper Coupons for May 2024
These Casper coupons can help you save big on your next bedding purchase from sheets, pillows, mattresses, and more.
-
Keeper Security Promo Codes for May 2024
Look through our Keeper Security promo codes to save on subscriptions to the online password manager and protect your details online for less.
-
TechRadar's story
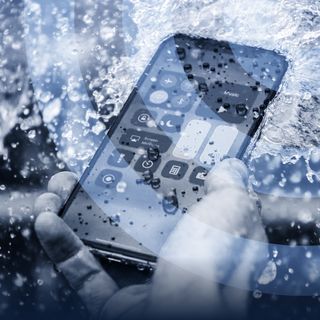
Our mission is unchanged
TechRadar was launched in January 2008 with the goal of helping regular people navigate the world of technology. It quickly grew to become the UK's biggest consumer technology site.
Expansions into the US and Australia followed in 2012 and we are now one of the biggest tech sites in the world.
- We've been covering tech since 2008
- 17 international editions from Mexico to New Zealand
- We're a globally respected brand worldwide
Software
All Software-
-
Do nearly all Indian men wear turbans? Generative AIs seem to think so, and it’s only the tip of the AI bias iceberg
Giving almost all Indian men turbans and other racist prompt results — can AI get past its bias stumbling block?
-
Logic Pro 2 is a reminder that Apple's AI ambitions aren't just about chatbots
The next-release of Logic Pro for iPad shows off Apple's AI chops with three new features.
-
Tired of ads in Windows 11? This free, straightforward third-party app might be just what you need to cut down the number of ads on your PC
Fed up with Windows 11 ads? OFGB could be your solution! It's a free app for disabling various ads across the operating system, providing a cleaner, hassle-free experience.
-
OpenAI is working on a new tool to help you spot AI-generated images and protect you from deep fakes
An AI image-spotting tool is in the works from OpenAI, and it's impressive in some respects, with a notable catch.
-
-
-
PlayStation Portal stock tracker - all the latest retailer restocks
We're tracking the PlayStation Portal stock situation for you, giving you the best advice on where to buy the PlayStation Portal remote play device.
-
Generative AI would help studios make games faster, says EA CEO - "there is a real hunger amongst our developers to get to this as quickly as possible"
Electronic Arts' CEO believes the use of advanced generative AI would make game development "more efficient".
-
Updated
Hellblade 2 release date and everything we know
Hellblade 2 is set to arrive this month, and while what we know is still fairlylimited, there's still a lot to unpack.
-
It took one day for Hades 2 early access to beat the first game's all-time peak player count on Steam
Hades 2 Early Access has already beat Hades' all-time peak player count on Steam.
-
Meet Your Experts
Between them, the TechRadar team have 300 years' experience in tech journalism. Here's why you should trust them.
-
-
Watch out — hackers can exploit this plugin to gain full control of your WordPress site
Vulnerable WordPress plugin allows hackers to create admin accounts and wreak havoc on the websites.
-
Tech enthusiast turns cheap QLC SSD into an expensive SLC one, massively improving endurance
Tech enthusiast turns cheap QLC SSD into an expensive SLC one, losing a large chunk of capacity but massively improving endurance.
-
Facebook will now let your business create entire ad campaigns using AI
New generative AI ad editing is coming to Facebook and Instagram as Meta rolls out new Verification tier subscriptions.
-
Mobile medical service DocGo confirms it suffered a major cyberattack
Hackers stole sensitive health-related data from an undisclosed number of customers.
-