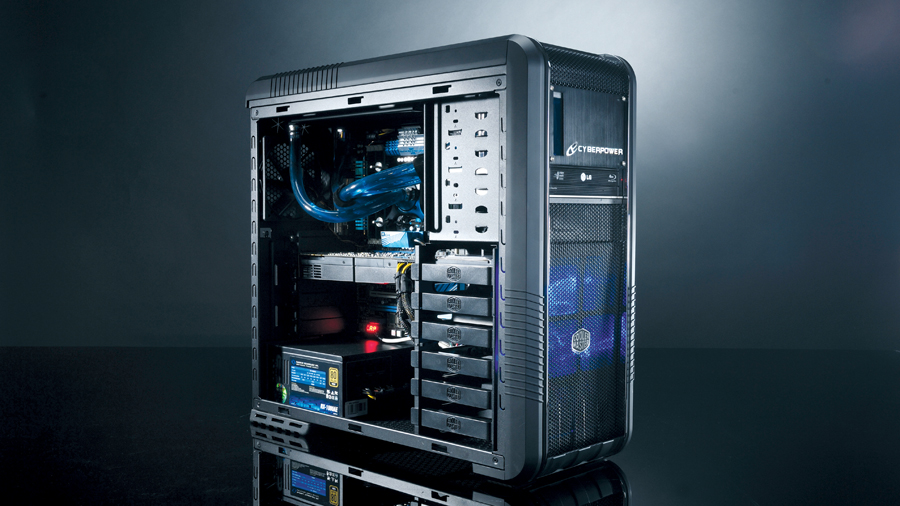
Call us sceptical, but we bet you didn't know 7,7,8,8-tetracyanoquinodimethane is contained within your PC. It's not nearly as well known as silicon, but today's motherboards wouldn't be nearly as reliable without it.
This is just the tip of the iceberg - PCs are built from a range of obscure materials, many of which are extremely expensive and difficult to mine or extract. Chances are you wouldn't be able to describe the properties of neodymium, ruthenium or gallium, but they all have a vital part to play in keeping your PC running smoothly.
Here we take a whistle stop tour of the PC, component by component, delving into the amazing substances that are used in its manufacture. We'll see something of their unique properties and why they're used, but we'll also investigate the materials themselves to see what they look like and where they come from.
We'll ponder what'll happen when supplies of the rarer elements run dry, investigate whether these chemicals pose a health risk, and reveal little-known and fascinating facts about these most mysterious of materials.
Of course, having these facts at your fingertips won't make you any more effective at improving your PC's performance or choosing your next upgrade, but you'll be off to a flying start when you're next asked, in a pub quiz, what links tantalum and niobium.
Silicon chips
So, silicon chips - and here we could include the processor, memory, GPU and the Southbridge chip - are made of silicon, right?
Well silicon is vitally important, but no chip made entirely from silicon would stand a chance of working. To look at a piece of silicon you'd think it was a metal, but if you were to drop it the chances are it would shatter, which isn't what you'd expect from a metal. With properties intermediate between metals and non-metals, silicon is part of a small group of chemical elements known as metalloids or semi-metals.
Get daily insight, inspiration and deals in your inbox
Sign up for breaking news, reviews, opinion, top tech deals, and more.
Semi-metallic is also how you'd describe silicon's electrical properties - it will conduct electricity, but not very well. However, by doping silicon - the technical term for adding a small amount of another element - its conductivity can be vastly improved.
This is the key to the operation of the transistor, the basic building block of all electronic circuits. To cut a long story short, doping with boron or arsenic turns the silicon into something called a p-type semiconductor, while doping with phosphorous or gallium creates an n-type semiconductor.
The reasons for this are too complex to explain here, but by combining n-type and p-type semiconductor materials you end up with a transistor, and by connecting enough of them together you can create a processor.
The transistors are connected using thin copper strips. A complicated chip will need several layers of copper tracks, so layers of insulating material must be used in between to prevent them shorting out.
The insulator is silicon dioxide - the same compound that makes up pure white sand. This can be produced easily by oxidising the surface of the silicon wafer as the chips are being manufactured.
The exotic mix of chemicals in a processor doesn't end here. Intel and IBM made the headlines a few years ago when they began using an element called hafnium to improve the performance of their chips. If you've not heard of this obscure element before, then you're in good company.
Suffice to say, it's a metal, and its close neighbours in the periodic table are the equally rare elements lutetium, tantalum, and the radioactive material rutherfordium. If you hanker after little-known facts, it's named after Hafnia, which is the Latin name for the Danish capital, Copenhagen, where it was discovered in 1923.
Worryingly, because of its use in the control rods of nuclear reactors, some experts have suggested that it will run out within 10 years at current rates of consumption. That's a long time in the world of semiconductors though, and we feel confident that an alternative - perhaps zirconium - will step up to the mark.
While the apparent scarcity of hafnium might suggest that it would be expensive, there's another element found in many processors that costs about 50 times more, gram for gram.
Gold is only used in very small quantities, but it's used as the plating on the pins or pads of most high-performance processors. The property that makes it such a good material for jewellery also makes it an excellent metal for plating components: as a fairly unreactive element, it doesn't tarnish due to reaction with the air.
This doesn't just affect its appearance - it means that the good electrical contact between the processor and its socket won't diminish over time.
If you consider yourself safety-conscious, you may have raised your eyebrows at the mention of one particular element used in semiconductor manufacturing. A hundred and fifty years ago, when the Cornish tin mining industry was in full swing, that element was a by-product of tin production, and was sold to America as a pesticide in the fight against the boll weevil beetle that was devastating the country's cotton crops.
The element in question is arsenic, a minor constituent of the tin ore cassiterite, and which was driven off by heat treatment in a calciner and condensed in a chamber called a labyrinth. Given the fact that the young boys who were employed to remove the arsenic from the labyrinth rarely lived beyond their early 20s, you'd be excused for questioning how sensible it is to put it in PCs.
You'll be relieved to hear that only small portions of a silicon chip are doped and, even then, concentrations measured in a few parts per million are all that's needed to provide those all important semiconductor properties. The bottom line is that integrated circuits contain no more than trace amounts of arsenic.
Hard disk platters
We all know that hard disks store data magnetically, but there are few similarities between them and the old DAT format used in the 1980s. Both use a head to read and write data to magnetic media, but there the similarity ends.
Magnetic disks use a much more efficient form of recording than is possible with a flexible tape, although that efficiency comes at a price. While magnetic tape is a simple, cheap strip of plastic film impregnated with iron oxide (rust), or more recently chromium dioxide, the platter of a hard disk drive is manufactured in a much more expensive multi-stage process that demands an extremely high degree of accuracy, and uses some interesting and esoteric materials.
The starting point is a plain disk, machined to high tolerances from a non-magnetic material. This way the magnetic properties can be accurately fine-tuned by adding thin layers of other materials.
The disk is typically made from either glass or metal. Aluminium is a good choice since it's light and non-magnetic, but hard disks need to be robust and aluminium isn't hard enough by itself. Instead, the material used is an alloy of aluminium and magnesium, plus small amounts of other elements like silicon, copper and zinc.
There are therefore five or more substances in the blank platter, although none of them are exactly rare or unusual - the more esoteric materials are used later.
The platter will spin at up to 7,200rpm, and the read/write head will hover millionths of a millimetre above it, so any unevenness would be catastrophic - the head would crash immediately. It isn't possible to polish aluminium alloy to the necessary smoothness, so the blank must be coated in a layer of a substance called NiP, which can take the high polish.
This material is an alloy of nickel and phosphorous. An alloy is usually thought of as a mixture of substances that shares their properties, but NiP couldn't be more different from its constituent parts.
Phosphorous is a non-metallic element, and a very reactive one at that. In its white elemental form, it will ignite spontaneously in air and burn fiercely. It's also highly toxic and glows in the dark. However, like nickel, NiP has all the properties of a metal and is relatively inert.
Strangely though, whereas nickel is magnetic, NiP is not. Most of the other materials on the platter are responsible for its magnetic properties. Iron is the most familiar magnetic material, but those you'll find in a hard disk are far more interesting. There are many magnetic (or, to be more accurate, ferromagnetic) metals, but those used in a hard drive are chosen for the ways in which they interact.
Since a more detailed explanation would take us into the intricacies of physics, we'll stay well clear of the 'why' and concentrate squarely on the 'what'. First there's something called the soft magnetic underlayer, which is made from an alloy of cobalt, nickel and iron.
In the highest performance disks, the soft magnetic underlayer is divided into two by a thin layer of the element ruthenium. Only a very small amount it is needed, which is just as well - as the 74th most abundant element on Earth (and there only are 90 naturally occurring elements), ruthenium is rarer than both gold and platinum.
Accounting for one part per billion of the Earth's crust, just 12 tonnes are produced annually, which is only enough to make a one-metre cube. Its name comes from Ruthenia, the 13th century Latin word for the ancient land of Rus, which comprised parts of current day Russia, Belarus, Ukraine, Slovakia and Poland.
The true recording layer is where we find the really expensive materials though, as we're now looking at an alloy of cobalt, chromium and platinum. Although platinum is more abundant than ruthenium, because it has so many more uses - mostly as a catalyst in the chemical industry and catalytic converters in cars - it's much more expensive. Currently it sells for $1,500 per Troy ounce, which works out as over $48,000 per kilogram.
Hard disk heads
Rare and expensive elements aren't only found on the platter, as we'll see when we look at that other important part of a hard disk drive - the read/write head. The head is attached to an arm assembly, which can move to access any of the concentric tracks of data on the platter.
An integral part of the arm is a wire coil that moves in a magnetic field whenever an electrical current is applied. That magnetic field is provided by a very powerful magnet, of which a major constituent is neodymium.
Neodymium looks much the same as any other metal, but it's unique in being the most magnetic of all the elements. In its raw form that property isn't particularly useful, since neodymium has such a low curie point - the temperature above which magnetism is lost - that anything containing a neodymium magnet would have to be refrigerated.
This is where those other metals come in. By mixing two parts of neodymium with 14 parts of iron and one part of boron, a combination of strong magnetism and a high curie point is achieved. And we really are talking about strong magnets - a neodymium-iron-boron magnet can lift over a thousand times it own weight.
This has given rise to safety concerns - if you ever try dismantling an old hard drive, make sure your fingers are well out of the way if the pair of neodymium magnets find themselves drawn together.
That's not all - although neodymium, iron and boron are all malleable, the alloy used in magnets is brittle, so if you do allow a pair of neodymium magnets to snap together from any distance, watch out for flying shards of alloy.