How GaN is changing the future of semiconductors
GaN is helping the semiconductor industry shake its reliance on silicon
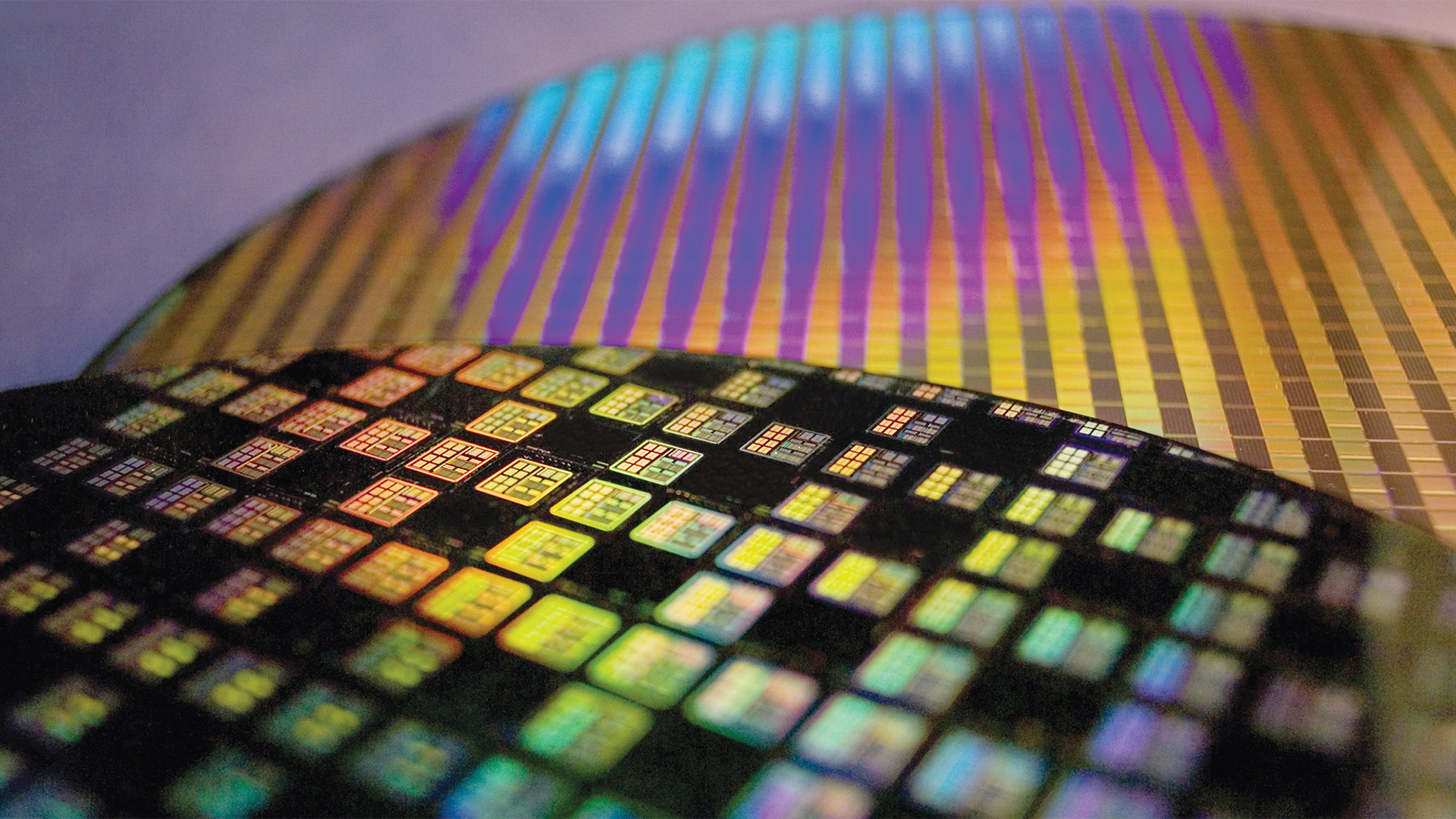
The global semiconductor shortage is delaying the production of everything from refrigerators and microwaves to gaming consoles and smartphones. Experts are saying it could take months for the industry to recover, but in reality the shortage is changing consumer electronics forever.
The industry has been reliant on silicon for decades, but the chip shortage is helping to make electronic devices more environmentally friendly, more efficient and smaller. More companies are turning to gallium nitride (GaN), because it is easier and faster to produce than silicon chips, among other benefits.
TechRadar Pro spoke to Stephen Oliver, VP Corporate Marketing and Investor Relations at Navitas Semiconductor, to find out how the shortage is impacting consumer electronics and shifting the industry away from silicon. Navitas supplies GaN chips to companies including Anker, Aukey, Belkin, Dell, Hyper, Lenovo, OPPO, RAVPower, Verizon and dozens of others.
Note that this article has been update with a new set of questions that Navitas answered recently on some industry trends in the GaN power supply market.
- Here's our list of the best business computers right now
- We've built a list of the best business laptops around
- Check out our list of the best business tablets out there
What is GaN and why is it so important?
Combining gallium (atomic number 31) and nitrogen (atomic number 7), gallium nitride (GaN) is a wide bandgap semiconductor material with a hard, hexagonal crystal structure. Bandgap is the energy needed to free an electron from its orbit around the nucleus and, at 3.4 eV, the bandgap of gallium nitride is over three times that of silicon, thus the designation ‘wide’ bandgap or WBG.
As bandgap determines the electric field that a material can withstand, the wider bandgap of gallium nitride enables the development of semiconductors with very short or narrow depletion regions, leading to device structures with very high carrier density. With much smaller transistors and shorter current paths, ultra-low resistance and capacitance is achieved, enabling speeds that are up to 100x faster.
The bottom line is that GaN technology can handle larger electric fields in a much smaller form factor than conventional silicon while delivering significantly faster switching. In addition, GaN technologies can operate at higher maximum temperatures than their silicon-based counterparts.
GaN is growing in importance because of its ability to offer significantly improved performance across a wide range of applications while reducing the energy and the physical space needed to deliver that performance when compared with conventional silicon technologies. In some applications where silicon as a power conversion platform has hit its physical limits, gallium nitride technologies are becoming essential, while in others the benefits of efficiency, switching speed, size and high-temperature operation combine to make GaN increasingly attractive.
As global need for energy increases, a move to GaN technology will help to meet demand while keeping carbon emissions to a minimum. Indeed, GaN design and integration has been shown to deliver next-generation power semiconductors with a carbon footprint ten times lower than older, slower silicon chips. To further support the case for GaN, it is estimated that a worldwide Si-to-GaN data center upgrade would reduce energy loss by 30-40%, which would translate as saving over 100 TWHr and 125 Million tons of CO2 emissions by 2030.
How is gallium made?
Gallium does not exist in elemental form in nature. It is typically derived as a by-product from the smelting of bauxite ore into aluminum and from the processing of sphalerite ore for zinc, so has a very low extraction and refinement carbon footprint.
How much does gallium cost?
Over 300 tons of gallium are produced every year, with over a million tons estimated in reserves around the world. As it is a processing by-product, it is relatively low cost, at around $300/kg which is 200x lower than gold, at around $60,000/kg.
Where is GaN used in electronics?
Gallium nitride has long been used in the production of LEDs and RF components but is now moving to mainstream acceptance in a growing number of power switching and conversion applications. Here GaN-based ICs can address demands to improve system performance and efficiency, save space and deliver reliable operation at higher temperatures.
Within phones and laptops GSM and Wi-Fi signals are transmitted and received using GaN RF devices, while the chargers and adaptors that power these devices increasingly incorporate GaN. Indeed, the largest market for power GaN is currently in mobile fast charging where GaN power ICs can enable three times faster charging in adaptors that are half the size and weight of slow, silicon-based designs. What’s more, for single-output chargers, GaN retail launch pricing is around half that of previous best-in-class silicon chargers and as much as three times lower in the case of multi-output chargers.
Gallium nitride power semiconductors are also being deployed in data center servers. As data center traffic accelerates, silicon’s ability to process power effectively and efficiently hits ‘physical material’ roadblocks. As a result, the old, slow, silicon chip is overtaken by high-speed gallium nitride ICs.
The consolidation of data center hardware, a new HVDC architecture approach and the proven reliability of mass-production, highly-integrated GaN power ICs enable major improvements in efficiency. Deploying GaN, therefore, represents another step towards carbon ‘Net-Zero’ goals for the data center industry.
In the automotive industry, gallium nitride is becoming the technology of choice for power conversion and battery charging in hybrid and electric vehicles. GaN-based power products can also increasingly be found in the inverters employed by solar power installations and in the power conversion schemes of motor drive and other industrial applications.
Why is GaN not impacted by the current chip shortage?
Silicon is a commodity, so manufacturers need to run at high percentage loading, 3-shifts, 24/7 to make money, with long lead times, and high capex to increase capacity. It is very difficult for silicon chip manufacturers to start and stop (due to Covid uncertainty), and there is very limited flexibility to recover from any stoppages
On the other hand, GaN has a very fast twelve-week lead time with spare capacity to ramp quickly, compared to 52+ weeks for some silicon devices. GaN can be produced more efficiently than silicon and the manufacturing process is more flexible resulting in GaN not being impacted like silicon.
Will GaN ever be a replacement for silicon?
Gallium Nitride (GaN) is a ‘wide bandgap’ (WBG) material, the bandgap being the energy required to free an electron from its orbit around the nucleus and allow it to move freely through the solid. This, in turn, determines the electric field that the solid is able to withstand.
Silicon (Si) has a bandgap of 1.1 eV, while GaN has a bandgap of 3.4 eV. As the WBG material allows high electric fields, depletion regions can be very short or narrow, so device structures can have higher carrier density and be packed very densely.
For example, a typical 650 V lateral GaN transistor can support over 800 V and has a drain drift region of 10-20 µm, or about 40-80 V/µm. This is substantially above the theoretical limit of silicon, which is around 20 V/µm. However, it is still well short of the bandgap limit of about 300 V/µm, leaving substantial room for generational improvements in lateral GaN devices in the future.
In device-level terms, the figure of merit derived from the product of normalized on resistance (RDS(ON)) and gate charge (QG) can be from five times to twenty times better than silicon, depending on implementation. By facilitating much smaller transistors and shorter current paths, ultra-low resistance and capacitance is achieved and switching speeds are up to one hundred times faster.
In order to fully exploit GaN power IC capability, the rest of the circuit must also be able to run effectively at higher frequencies. In recent years, control ICs have been introduced to take switching frequencies from 65-100 kHz up to 1 MHz+, with new controllers in development. Microcontrollers and digital signal processors (DSPs) can also be used to implement today’s soft switching circuit topologies, while a broad range of magnetic materials that are optimized for the 1-2 MHz range are now available.
GaN power ICs combine frequency, density, and efficiency advantages in half-bridge topologies such as active clamp flyback, totem-pole PFC and LLC. With a change from hard- to soft-switching topologies, the general loss equation for a primary FET can be minimized, leading to improved efficiency at 10x higher frequencies.
GaN is processed using 250-350 nm CMOS equipment for relatively-large feature sizes for power processing. CPUs, GPUs use silicon at ~1V – and use sub-10 nm process equipment to get very-fine feature sizes for digital processing. So – the optimal point is to use GaN for ‘power conversion’, and silicon for ‘data processing’.
What does the future hold for GaN?
With record-breaking performance, gallium nitride power ICs are the catalyst for a second revolution in power electronics. GaN currently covers a device voltage range from 80-900V – with ongoing research work to take it lower, and higher.
What is fuelling the rapid rise in power charging capacities?
For mobile markets, driven by larger screens and increased features & functions, battery sizes (mAhr) have increased 10x in three years, while users expect to charge devices in a minimum time. New gallium nitride (GaN) semiconductor technology is fundamental to this rapid rise of power charging capacities as they can offer significantly improved performance across a wide range of applications while reducing the energy and the physical space needed to deliver that performance when compared with conventional silicon technologies. GaN ICs run up to 20x faster than old, slow silicon (Si) chips, and enable up to 3x more power or 3x faster charging in half the size & weight.
Additionally, there is a global need for greener energy. High-efficiency, high-speed applications using GaN power ICs are smaller, lighter and use less material and less energy than silicon systems. Each clean, green GaN power IC shipped saves 4 kg CO2. GaN could save up to 2.6 Gtons/year CO2 – equivalent to emissions from 650 coal-fired power stations
There's a belief that fast charging could damage batteries, how true is that these days?
The Universal Serial Bus Power Delivery (USB-PD) protocol is a communication and protection loop between the charger and the driven device, whether phone, tablet, laptop or earbuds. For example, a smartphone will inform the charger how much power and at what voltage it is to deliver – and this is safely within the design limits of the battery technology. At the same time, new battery technologies like graphene lithium ion allow faster charging, with cool operation and long lifetime. For example the new Realme Neo GT 3 uses a 150W ultra-fast charger to power a 4,500mAh battery from 0-100% in only 9 minutes – while keeping the battery at a low temperature. The charging protocol also enables a high current (charge rate) when the battery is dead / low and then reduces the current as the battery approaches full charge.
Sign up to the TechRadar Pro newsletter to get all the top news, opinion, features and guidance your business needs to succeed!
Could we see this type of technology come to PC and laptops where big, fat power supplies are still the norm?
Certainly, GaN chargers are already making their way into laptop chargers, for example, the LG gram, Dell Latitude, Lenovo Xiaoxin and Yoga Laptops. For example, the new Dell 60W charger is an ‘in-box’ optional upgrade from a legacy silicon-based charger. Measuring only 66 x 55 x 22 mm (94 cc) and weighing only 175 g, the charger is 50% smaller and 25% lighter than the previous silicon-based generation. It should also be noted that the ‘green’ benefits of GaN enable the OEMs to achieve their ‘netzero’ goals – for example, a GaN-based 65W adapter has a 30% lower CO2 footprint than for legacy silicon.
Where's the limit and what are the obstacles to faster or even quasi-instantaneous charging?
The Universal Serial Bus Power Delivery (USB-PD) protocol has an extended power range, now up to 240W which allows interoperability, speeding market acceptance. OPPO has revealed a platform capable of up to 240W, so an ‘instant-charge’ smartphone could be on the horizon.
Would you roll that out to cars as well at some point? or other products that require fast charging?
Absolutely, the same 3x faster charging can apply to any battery-based application – reducing range and charge-time anxiety. GaN-based on-board chargers (OBCs) are estimated to charge 3x faster with up to 70% energy savings compared to legacy silicon solutions. GaN OBCs, DC-DC converters and traction inverters are estimated to extend EV range or reduce battery costs by 5%, and to accelerate the adoption of EVs worldwide by 3 years. An EV-upgrade to GaN could pull-in EV adoption worldwide by three years and is estimated to reduce road-sector CO2 emissions by 20%/year by 2050, the target of the Paris Accord. This is a critical area for all of us.
- We've built a list of the best mobile workstations available
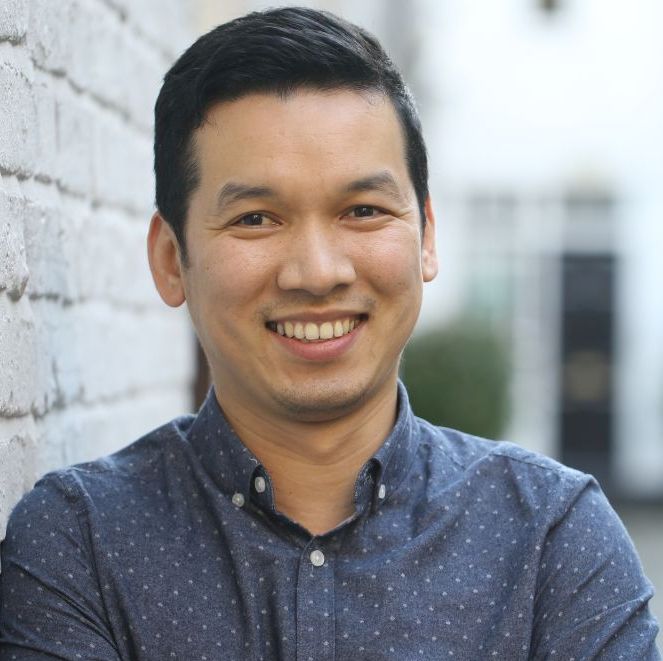
Désiré has been musing and writing about technology during a career spanning four decades. He dabbled in website builders and web hosting when DHTML and frames were in vogue and started narrating about the impact of technology on society just before the start of the Y2K hysteria at the turn of the last millennium.